Raman Spectroscopy vs Fourier Transform IR Spectroscopy
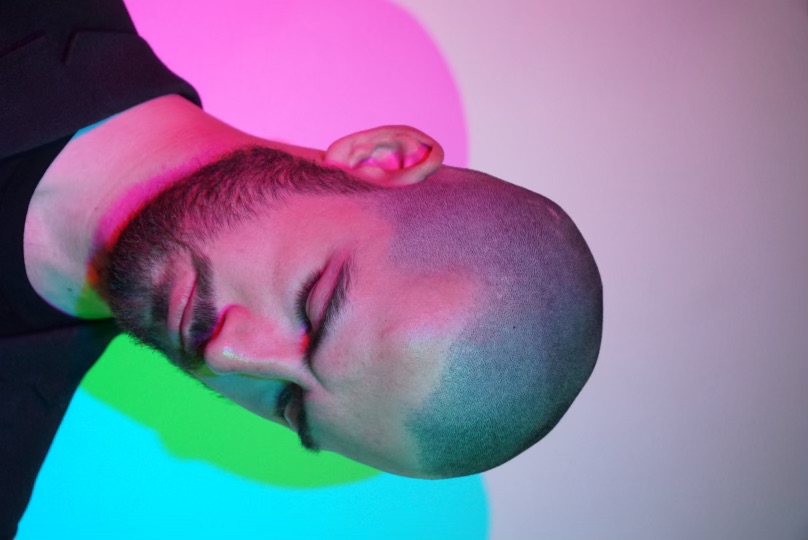
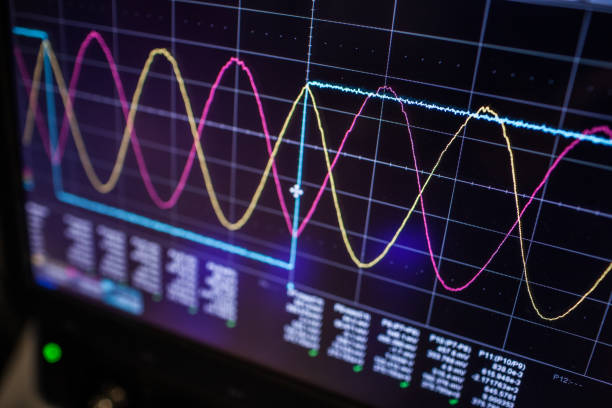
I recently watched Dr Doug Baney’s inaugural lecture, speaking at the University of Edinburgh’s School of Engineering. Doug Baney has been a key figure for the School of Engineering, providing its students with the best of KeySight’s test equipment to improve the lab’s capabilities. Baney’s inaugural lecture focussed on his rich career in lasers and developing laser interferometers.
Within the rich history of interferometry, from Michaelson’s measurement of the ether to the discovery of gravitational waves, one of the examples Baney presented was a Fourier Transform IR Spectrometer.
An intriguing device with simple but effective qualities, the system works by sending a monochromatic laser beam through a beam splitter which then reflects off a translation mirror. The resulting beam is interfered with either destructively or constructively, depending on the position of the mirror, and is then directed towards a laser-scattering chemical sample. The chemical, with its unique vibrational fingerprint, will absorb some of the laser light and induce vibration of the chemical bonds. This change in intensity is finally detected and processed digitally. As the mirror moves back and forth, the laser light produced varies in intensity in a sinusoidal pattern. The wavelength of the light is then changed and another sinusoid is produced. If we then take a Fourier transform of these sinusoids, a spectrum of different frequencies is produced, with the intensity of the Fourier transform proportional to the coherence of the wave. The coherence of the scattered light from the chemical varies due to the vibrational modes of the chemical.
This technique increases the spectral resolution of the spectrum, presenting finer details of vibrational modes. The spectral resolution can be multiplied depending on how fine you can tune the displacement of your mirror. Since the Fourier transforms invert the dimensionality of the processed interferogram ( [L] to [L-1]), a 0.25cm displaced mirror can result in a 4 cm-1 resolution. In this particular way, the resolution dependence has been transferred from the spectrometer plate to the scanning mirror alignment.
Diagram of Fourier Transform IR Spectrometer, showing oscillating intensity, combined into a Fourier transform where the intensity is proportional to the wave’s coherence.
An advantage of this method is the simultaneous collection of information from all wavelengths. This leads to a higher signal-to-noise ratio for a given scan time in observations limited by a fixed detector noise contribution. This advantage is particularly noticeable in the thermal infrared spectral region, where photodetectors are restricted by generation-recombination noise. If a spectrum has m resolution elements, the increase in signal-to-noise ratio is proportional to the square root of m. Alternatively, it allows for a shorter scan time for a given resolution. In practice, multiple scans are often averaged to increase the signal-to-noise ratio by the square root of the number of scans.
One of the key differences compared to Raman spectroscopy is the spectrum produced. In IR spectroscopy, an absorption spectrum creates a deep valley, whereas a Raman spectrum would excite a corresponding small peak. Likewise, a large Raman peak corresponds to a small (sometimes unnoticeable) IR absorption.
Raman (green) and IR Absorption spectrums of mesitylene and indene.
(a) IR absorption and (b) Raman spectrums of unknown chemical
Combined Raman and IR vibrational mode print of unknown chemical
This difference is explained by thinking of the molecule as a set of connected springs and masses. For a heavy set of masses, and a strong bond, it takes a lot of energy to stimulate a vibration, thus producing a small Raman peak. However, this complex is very good at absorbing radiation, producing a deep IR absorption. Likewise, a lightweight atomic-bond complex will be very easy to vibrate and re-emit high-intensity inelastic light but will not undergo absorption.
The best system for chemical fingerprinting incorporates both Raman and IR spectroscopy: where Raman detects the light-weight molecules and IR detects the heavier absorbing molecules. Once superimposed both spectrums are on a Raman shift scale, one can have a series of lines to enhance the dimensionality of your data sample and utilise both methods.
If you enjoyed this article and would like to hear Dr Doug Baney's Inaugural Lecturer as an Honorary Visiting Professor for the University of Edinburgh, see the link below: https://www.youtube.com/watch?v=KrwDnGUwY-c
Subscribe to my newsletter
Read articles from Clark Gray directly inside your inbox. Subscribe to the newsletter, and don't miss out.
Written by
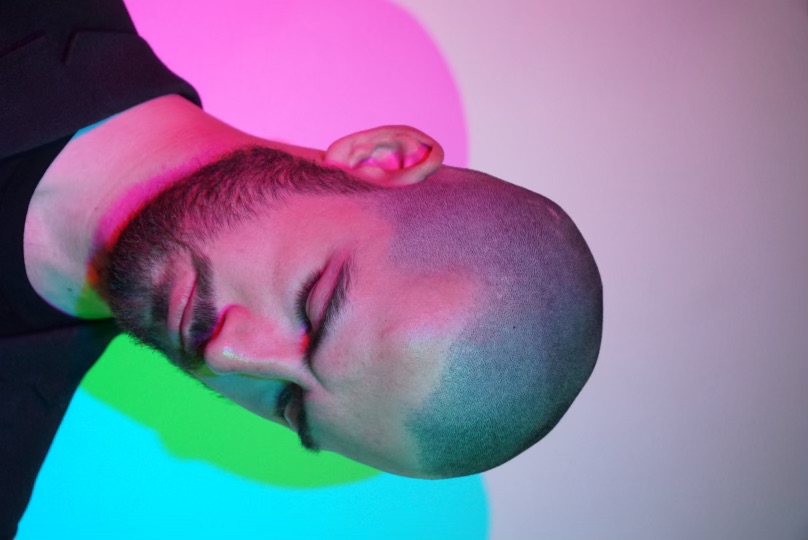