Virus-Like Particles (VLPs)
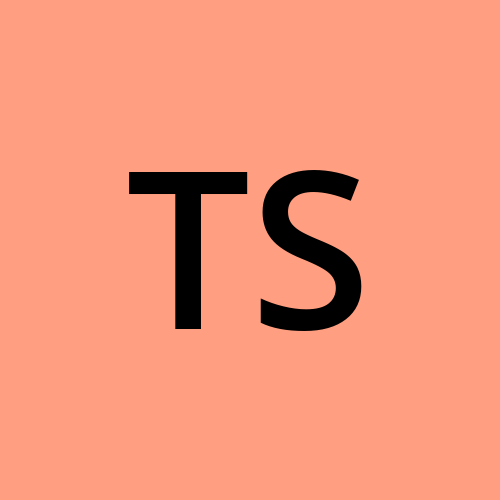
Introduction
Virus-like particles (VLPs) are hollow protein particles that are similar in shape to natural virus particles and are self-assembled by one or more structural proteins of viruses. The diameter of VLPs is about 20~200nm, which has a regular spatial structure and superior biocompatibility. The conformation of VLPs is very similar to that of real viruses, but it does not contain the nucleic acid required for virus replication and has no infective ability1. Therefore, it does not have potential risks such as incomplete inactivation of virus, virus genome exchange and recombination, and virus atavism.
Structural Classification of VLPs
Spontaneous polymerization of different viral capsid proteins can produce VLPs with geometric symmetry, usually in the form of icosahedral, spherical or rod-shaped structures, depending on which virus they come from. VLPs can be classified according to the complexity of their structure, and capsid proteins can be arranged in one, two, or three layers.
Enveloped VLPs are also subdivided into monolayer, bilayer, and multilayered internal structures, which lie beneath the lipid envelope. Enveloped VLPs acquire lipid membranes from cells that express the assembly and budding of VLPs. One or more anchors of glycoproteins can be inserted into the lipid membrane. These glycoproteins are usually the main target antigens detected by the immune system for the production of neutralizing antibodies.
Fig. 1 Schematic diagram of enveloped VLP production (Kushnir, N.; et al. 2012)
Non-enveloped VLPs are further classified as single or multi-capsid protein VLPs and also as single-layer, double-layer, and triple-layer VLPs. The simplest available non-enveloped model of VLP consists of single capsid VLP structure like human papillomavirus (HPV) VLP vaccines2. These simple VLPs are composed of a single capsid protein that can be expressed in both eukaryotic and prokaryotic systems.
Fig. 2 Schematic diagram of non-enveloped VLP production (Kushnir, N.; et al. 2012)
Expression System
- E. coli
As the most widely used expression system for the preparation of recombinant proteins, the E. coli expression system is not widely used for the preparation of VLPs, mainly due to the lack of a post-translational modification system similar to that of mammalian cells. However, it has been found that E.coli can express some acapsular VLPs. The first VLPs expressed by E. coli in the world was the hepatitis E vaccine.
- Yeast
Merck's Gardasil® for the prevention of early cervical and vaginal cancer and Recombivax HB® for the treatment of hepatitis B are both manufactured through this expression system4. Similar to the expression system of Escherichia coli, the posttranslational modification function of yeast, especially the deficiency of protein glycosylation, limits its application in VLPs production. The system is also commonly used to produce acapsular VLPs.
- Insect cell
The most commonly used insect cell expression system is the insect-baculovirus expression system, through which GlaxoSmithKline produced vaccines containing VLPs of HPV types 16 and 18. The insect cell expression system can perform the function of post-translational modification of proteins, glycosylate target proteins, and accumulate a large amount of foreign proteins without animal pathogens. However, since the target protein is co-expressed with baculovirus particles, it is easy to be contaminated. Thus, purification of VLPs is often an issue when using insect-baculovirus expression systems.
- Mammalian cell
The most important feature of mammalian cell expression systems is that they can correctly and effectively recognize the signals of eukaryotic protein synthesis, processing, and secretion, especially that they can accurately complete post-translational modifications such as glycosylation and phosphorylation. The resulting proteins have complete structure and function, so they are widely used in the production and research of therapeutic recombinant proteins.
- Plants cell
Plants expression systems have several advantages over conventional approaches, including high expression levels of up to 80% total soluble protein, low refining costs, and high-performance expression processing. Using MagnICON and CPMV-HT technologies, plant-based vaccines have emerged as a versatile and promising platform enabling protein production at less than US $50 per gram for the production of several proteins and antibodies for the human and veterinary pharmaceutical industries5.
- Cell-free system
Cell-free protein synthesis (CFPS) systems provide an additional option for the in vitro expression express the recombinant proteins for VLPs production6. CFPS systems have many advantages compared to cell-based protein expression platforms such as time-saving, high yield of proteins, limited cellular contaminants and the option of generating VLPs containing unnatural amino acids (UAAs) or toxic protein intermediates.
Application
- Prophylactic vaccine
VLPs is highly immunogenic and can induce humoral and cellular immune responses. Currently, a variety of VLPs-based subunit vaccines have been developed, the latest of which is the vaccine against novel coronavirus (SARS-CoV-2). In 2020, Medicago expressed the novel Coronavirus S protein through the plant expression system7.
- Therapeutic vaccine
In addition to immunity against pathogenic microorganisms, VLPs can also overcome the tolerance of the immune system by inducing specific antibodies against endogenous molecules of chronic diseases in the body. Therefore, chimeric VLPs is used as a therapeutic vaccine.
Fig. 3 Overview of VLP-based vaccine expression, purifcation and formulation (Nooraei, S.; et al. 2021)
- Drug/antigen delivery carrier
Antigen-presenting cells (APCs) can usually recognize antigens with a diameter of 20 nm~3μm, and the optimal diameter for dendritic cells to recognize antigens is 40 nm, which is exactly consistent with the size range of VLPs. This indicates that VLPs can be used as an antigen delivery carrier, carry other antigens, be captured by APCs, and activate immune cells to activate the immune system. VLPs has clear structure, suitable particle size, superior biocompatibility, and specific targeting, so it is a reliable biological carrier for drug transport. For example, the HBV virus naturally infects the liver, so HBV VLPs can specifically target liver cells. Similarly, rotavirus VLPs can target drug delivery to intestinal tissue8.
- Diagnosis
VLPs have a large surface area and are protein cages with relatively large cavities that can provide storage space for biomolecules. For example, the encapsulation of fluorescent dyes, magnetic nanoparticles and contrast agents (such as gadolinium ion tracers) or chelated metals in the internal cavity of VLPs is widely used in medical diagnosis and treatment fields such as magnetic resonance imaging or angiography9.
- Models for immune research
VLPs is similar to viruses in shape, size and structure. Under low biosafety levels, it can be used to study the infection and antibody neutralization of highly pathogenic viruses. Moreover, VLPs as a carrier can carry foreign virus genes and study the replication and original transcription of virus genes at the same time. On the other hand, VLPs can be used to study how APCs, especially dendritic cells, recognize, present and process pathogens.
References
Bai, B.; et al. Virus-like particles of SARS-like coronavirus formed by membrane proteins from different origins demonstrate stimulating activity in human dendritic cells. PLoS One. 2008 Jul 16;3(7):e2685.
Chen, XS.; et al. Papillomavirus capsid protein expression in Escherichia coli: purification and assembly of HPV11 and HPV16 L1. J Mol Biol. 2001;307(1):173-182.
Nooraei, S.; et al. Virus-like particles: preparation, immunogenicity and their roles as nanovaccines and drug nanocarriers. J Nanobiotechnology. 2021 Feb 25;19(1):59.
Kushnir, N.; et al. Virus-like particles as a highly efficient vaccine platform: diversity of targets and production systems and advances in clinical development. Vaccine. 2012 Dec 17;31(1):58-83.
Gleba, Y.; et al. Magnifection—a new platform for expressing recombinant vaccines in plants. Vaccine. 2005;23(17-18):2042-2048.
Glass, PJ.; et al. Norwalk virus open reading frame 3 encodes a minor structural protein. J Virol. 2000;74(14):6581-6591.
Batty, CJ.; et al. Vaccine formulations in clinical development for the prevention of severe acute respiratory syndrome coronavirus 2 infection. Adv Drug Deliv Rev. 2021 Feb;169:168-189.
Zdanowicz, M.; Chroboczek J. Virus-like particles as drug delivery vectors. Acta Biochim Pol. 2016;63(3):469-73.
Manchester, M.; Singh, P. Virus-based nanoparticles (VNPs): platform technologies for diagnostic imaging. Adv Drug Deliv Rev. 2006 Dec 1;58(14):1505-22.
Subscribe to my newsletter
Read articles from Thomas Schmitt directly inside your inbox. Subscribe to the newsletter, and don't miss out.
Written by
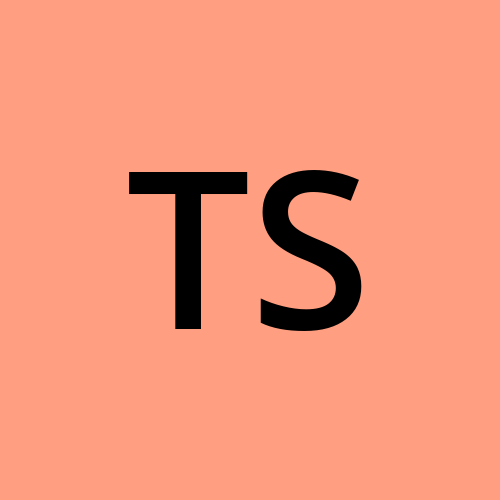