Why Don’t We See a Ball Acting Like a Wave?
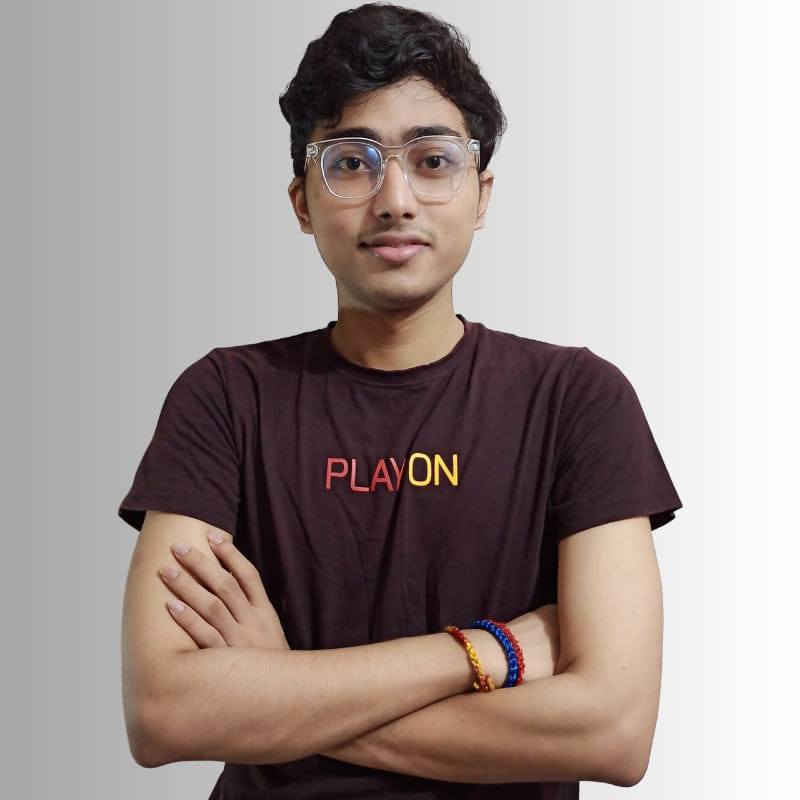
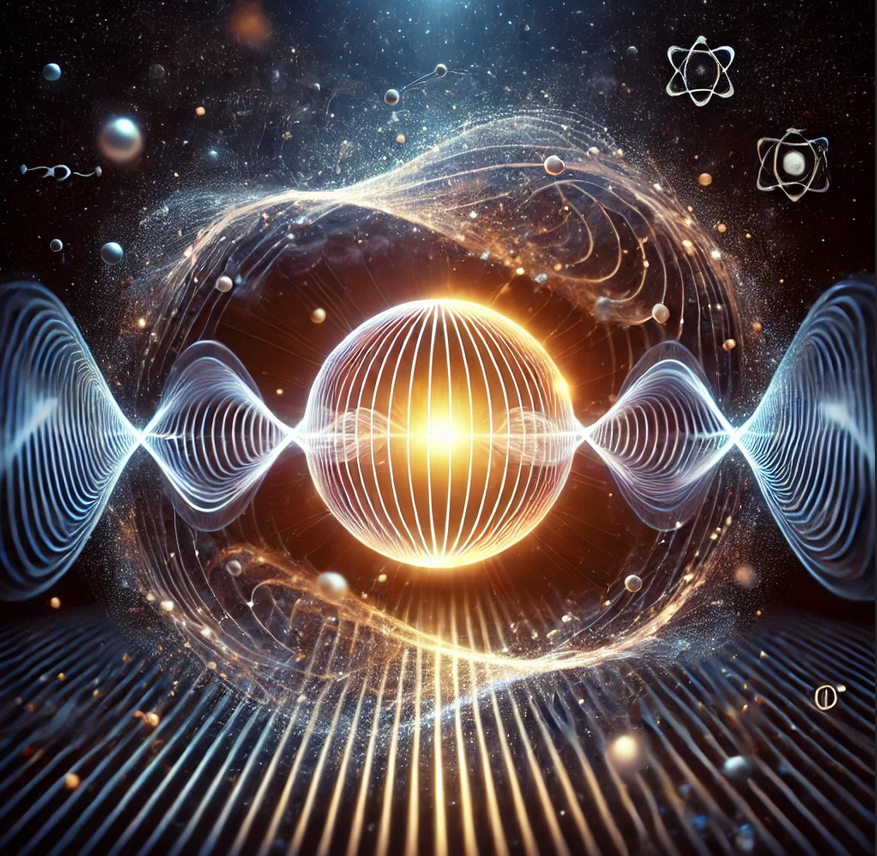
The dual nature of light, behaving as both a wave and a particle, has puzzled scientists for decades. This wave-particle duality, discovered through experiments like the famous double-slit experiment, shows that light, and all matter, exhibit both properties. But if this is true, why don’t we observe everyday objects, like a ball, acting as waves?
At the quantum level, everything, from photons of light to electrons and even larger objects, has a dual nature. According to quantum theory, every particle has an associated wavelength, known as the de Broglie wavelength. The smaller the object, the more prominent its wave-like behavior. For example, electrons, which are incredibly tiny, can easily show wave-like behavior in experiments.
But as objects get larger, their wavelength becomes smaller and smaller. For something like a ball, the wavelength is so minuscule that any wave-like behavior is effectively invisible to us. This is why we never see a ball "spread out" like a wave; it behaves more like a particle in our everyday experience.
In quantum mechanics, particles exist in superposition – they can be in multiple states at once. However, this superposition only holds true when particles are in coherence, meaning their wave functions are synchronized. Think of it like a perfectly choreographed dance: as long as the particles are in sync, their wave-like behavior can be observed.
As objects increase in size and complexity, synchronization becomes harder to maintain. This is due to a phenomenon called decoherence, which occurs when particles in a system interact with their environment. In the case of larger objects like a ball, there are so many particles, all interacting with each other and their surroundings, that they quickly lose synchronization. As a result, the wave-like properties disappear, leaving only the familiar particle-like behavior we observe in the classical world.
The quantum world is strange and often counterintuitive, where particles can behave like waves, and objects can exist in multiple states. However, the effects of quantum behavior, such as superposition and interference, become less noticeable as we move to larger scales. This is because the wavelength of an object is inversely proportional to its mass and velocity. For a photon, which has no mass, its wave-like nature is very evident. For a ball, with significant mass, its wavelength is so small that its wave nature is essentially undetectable.
While large objects like balls don’t show wave-like behavior, quantum phenomena like tunneling offer a glimpse into how the quantum world can affect larger objects. In quantum tunneling, particles can “tunnel” through barriers that they shouldn’t be able to cross based on classical physics. This wave-like behavior allows particles to appear in places they shouldn’t, defying our classical understanding of particles being in one place at one time.
Although we don’t see quantum tunneling in everyday objects, this phenomenon happens on an atomic scale. The same quantum principles that allow light to act as both a wave and a particle are at play here, showing us how strange and wondrous the quantum world can be.
A key concept in quantum behavior is synchronization. When quantum particles like electrons or photons are in sync, they can behave as waves, exhibiting interference patterns and other quantum effects. However, as the number of particles increases, maintaining synchronization becomes impossible due to constant interactions with their environment. This desynchronization, or decoherence, marks the transition from the quantum world of waves to the classical world of particles.
In a way, you could think of synchronization as the “glue” that holds quantum effects together. As long as particles remain coherent, they can act like waves. But as soon as decoherence occurs, wave-like behavior disappears, and the classical particle-like nature dominates.
So, why don’t we see a ball acting like a wave? The answer lies in the quantum world’s delicate balance of synchronization and decoherence. While all objects, regardless of size, have wave-like properties, the effects become less noticeable as the object gets larger and its wavelength decreases. Decoherence, caused by constant interactions with the environment, quickly desynchronizes large objects, leaving us with the classical world of particles we’re familiar with.
The wave-particle duality that governs light and other small particles fades as we move to larger scales, but it never truly disappears. Quantum mechanics teaches us that the universe is far stranger than it appears at first glance, and even though we don’t see a ball acting like a wave, the underlying quantum principles are still at play.
Subscribe to my newsletter
Read articles from Swadhin Roy Sunny directly inside your inbox. Subscribe to the newsletter, and don't miss out.
Written by
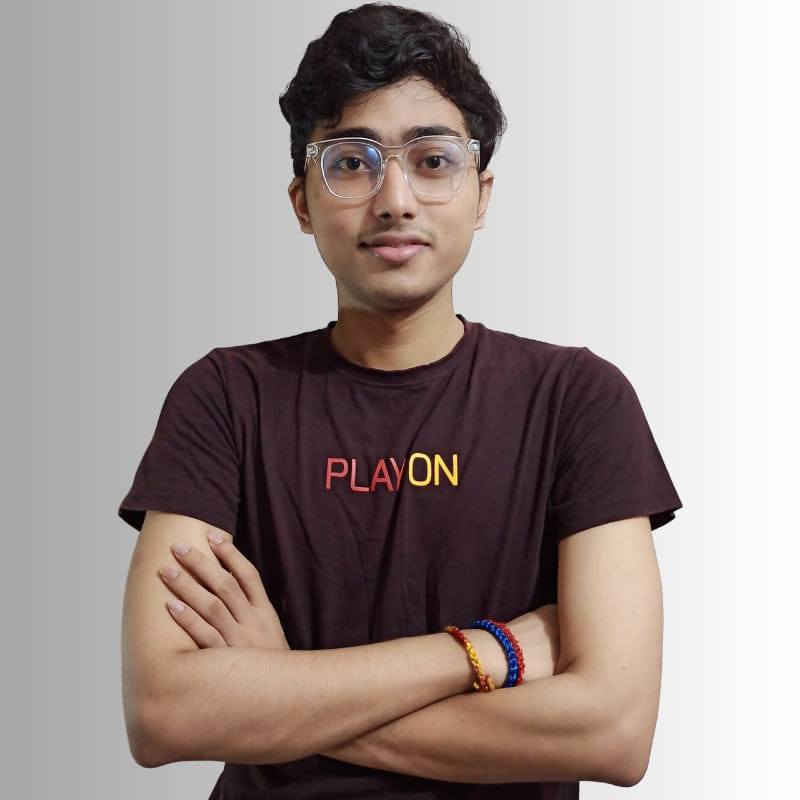
Swadhin Roy Sunny
Swadhin Roy Sunny
I am Swadhin Roy Sunny, a highly motivated STEM enthusiast, mental health advocate, and young researcher. As an international award-winning student, I am dedicated to making a positive impact through science and technology. I founded Monobadh, a non-profit organization focused on mental health awareness, and I am also a content creator on YouTube, sharing my passion for learning and discovery. As a young entrepreneur, I am committed to fostering innovation and growth. I enjoy writing about travel, life philosophy, and physics, and I am always eager to connect with like-minded individuals and professionals.