Quantum Computing's Impact on Current Encryption Methods

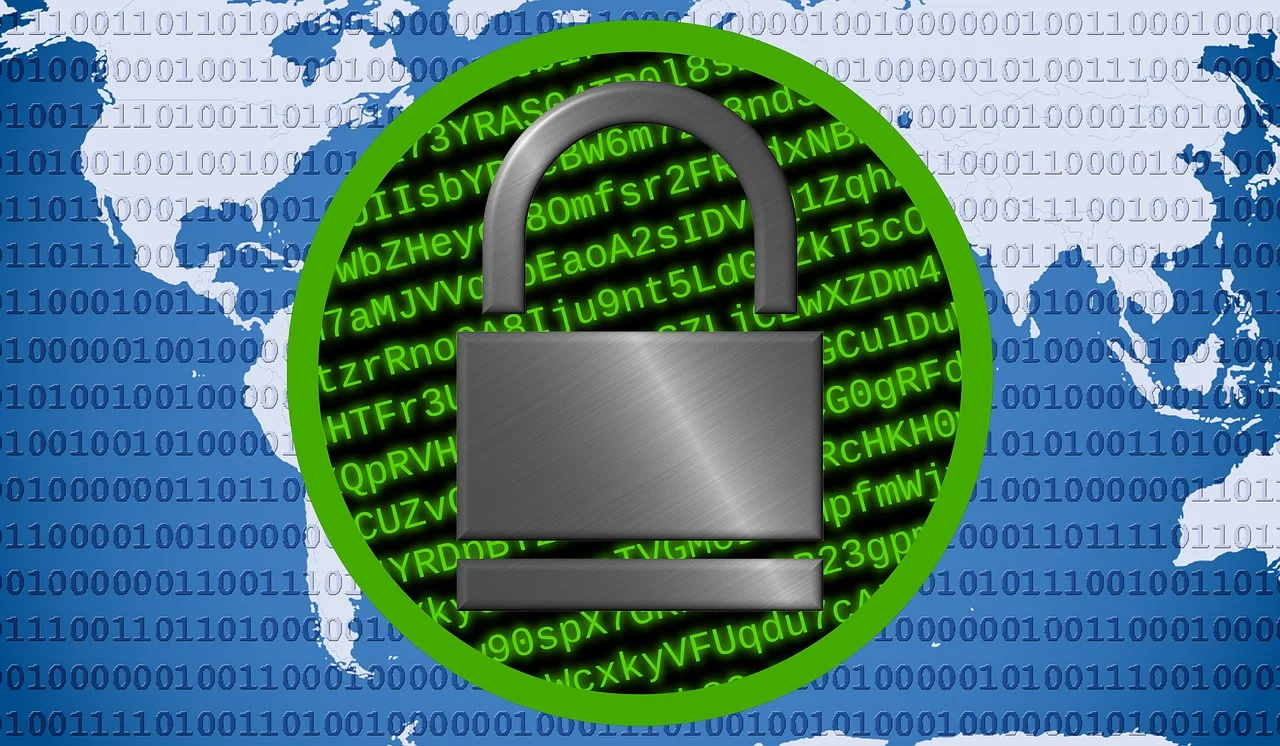
Introduction
Quantum computing is a wonder of modern times, still in the embryonic stage of development and assurance of computation speed unparalleled by classical computers. While this may open new avenues, it is also one of the most dangerous technological advancements to cybersecurity practices as we know them, especially encryption. For all practical purposes, encryption is the bedrock on which modern data security rests—the thing that enables everything from secure online transactions to personal communication privacy. However, many of today's widely used techniques may become vulnerable with the advent of quantum computers.
Some of the implications quantum computing has for current encryption methods are discussed in this article by showing the vulnerabilities this emerging technology exposes and the steps taken to secure the post-quantum world.
Understanding Quantum Computing
Basically, to understand how quantum computing can disrupt encryption, we should first understand what makes quantum computers different from their classical counterpart. While classical computers operate with bits, which is the smallest unit of information, and can take the value 0 or the value 1, quantum computers operate on qubits that can represent not just 0 or 1 but both at the same time because of the principle called superposition. This, in effect, makes it possible for quantum computers to process exponentially more information than classical systems.
Entanglement is another important aspect of quantum mechanics that allows qubits that are entangled to stay connected in such a way that the state of one instantly impacts the state of another, irrespective of the distance between them. These properties allow quantum computers to solve problems in ways that would normally take classical computers thousands, if not millions, of years to compute.
While the development of fully functional quantum computers is still in the development process, the pace of improvement is accelerating. Practical quantum computers capable of breaking classical encryption could be less than ten years away, according to expert estimates.
Overview of Current Encryption Methods
Digital information must be safeguarded, and for that purpose, encryption is very essential. It is based on mathematical algorithms that change the data to unreadable formats without the use of a decrypting key. Basically, there exist two types of encryption in common practice: symmetric encryption and asymmetric encryption.
Symmetric Encryption: This is symmetric because it requires just one key for encryption and decryption. A very common example of this is the Advanced Encryption Standard (AES), which has wide use in securing sensitive data because of its computational efficiency.
Asymmetric Encryption: Unlike symmetric encryption, in this process, two keys are being used—a public key for encryption and a private key for decryption. One of the common algorithms includes RSA, or Rivest-Shamir-Adleman, and ECC, or Elliptic Curve Cryptography. These methods have special use in securing communication between parties without previously shared keys.
These encryption methods are designed to be computationally hard to break, leveraging difficult mathematical problems such as factoring large numbers or computing discrete logarithms—things that classical computers cannot do in a feasible amount of time.
Why Quantum Computing Threatens Current Encryption
This enormous power of quantum computers threatens the existing techniques of encryption, more precisely asymmetrical encryption. The reason for this threat includes quantum algorithms that can solve factorization problems and discrete logarithms in polynomial time—both of which RSA and ECC rely on for security. With Shor's algorithm, a quantum computer could break RSA encryption in just a couple of seconds and render it obsolete.
For symmetric encryption schemes like AES, quantum computers are less of an imminent threat; the danger is nevertheless serious. In the sense that it does not threaten the security directly, quantum computers are not directly threatening symmetric encryption. However, this is where it gets interesting: a quantum computer can vastly decrease the time taken to carry out a brute-force attack on an encryption key. There is, for example, a quantum algorithm called Grover's Algorithm that could reduce the time to break an AES key by half. That would be interpreted to mean that, while AES encryption would not be broken outright, longer key sizes—for example, 256-bit keys—would be needed to maintain sufficient security.
In practical reality, the effects of quantum attacks on encryption can be really bad: sensitive financial data, personal information, and communications now protected with RSA or ECC might be decrypted, leading to wholesale data breaches and privacy violations.
Post-Quantum Cryptography (PQC)
Realizing that quantum computing will totally break most, if not all, of today's encryption, researchers are now developing what is called post-quantum cryptography that is resistant against both classical and quantum computers. The goal of PQC is to develop new cryptographic schemes that avoid quantum attacks but remain efficient on classical computers.
Organizations like the National Institute of Standards and Technology (NIST) work to standardize the PQC algorithms. NIST is working on the selection of new algorithms, a replacement for RSA and ECC, by focusing on mathematical problems that no quantum computer can solve in good time, like lattice-based cryptography and hash-based cryptography.
Among the promising PQC algorithms are:
Lattice-based cryptography: This would be based on the difficulty of problems related to lattices and is also resistant to both classical and quantum attacks.
Hash-based cryptography: this is such a kind of cryptography where hash functions would be in a leading role to generate secure signatures.
Multivariate polynomial cryptography: This is based upon solving systems of multivariate equations.
Yet, against this strong research development, there exist some stumbling blocks. Usually, the PQC requires higher computational resources, which fact makes them slower and heavier on bandwidth as compared to the encryption being presently used. Clearly, adapting these algorithms to real-world applications and integrating them with the existing system will require careful planning and innovation.
Quantum-Safe Encryption: Current Developments
To this end, researchers and organizations are developing quantum computers that would deal with the looming threat in order to protect sensitive data in a quantum-powered future. One such approach involves what is termed "Quantum-Safe Encryption," focused on adapting existing systems or designing new ones to resist quantum attacks.
Several technology giants, cybersecurity firms, and governments are deeply investing in quantum-resistant encryption techniques. These are not merely theoretical, as many are already building the infrastructures necessary, which, in turn, would protect sensitive information in a post-quantum world. Examples include Google, IBM, and Microsoft experimenting with post-quantum algorithms as part of their encryption suites to be ready in case quantum computers become viable.
Of the most promising developments in quantum-safe encryption, perhaps the most salient is quantum key distribution. Unlike other cryptographic methods that rely on highly complex mathematical problems as their security basis, QKD relies on the principles of quantum mechanics itself to securely exchange encryption keys across parties. The beauty of QKD lies in the fact that any attempt to eavesdrop or intercept the communication will disturb the quantum state, hence warning users of a potential breach. This theoretically guarantees a safe key exchange from both classical and quantum attacks.
However, QKD itself has various limitations. It indeed requires very special hardware for implementation and infrastructure, like quantum channels for key distribution, and cannot be scaled up over long distances without optical networks. This makes it less practical compared to software-based post-quantum cryptographic solutions for everyday use in conditions of widespread applicability.
The Road Ahead: Preparation for a Quantum Future
As quantum computing is getting closer to reality, so is the reality of such challenges that businesses and cybersecurity professionals alike will have to face. The transition from current cryptographic systems to quantum-safe solutions will need very careful planning, major investment, and a phased approach to make sure that the impact on operations is minimal.
Some of the major strategies to help an organization prepare for a quantum future include:
1. Quantify Current Cryptographic Infrastructure: Firms should perform a deep audit of the existing cryptographic setups in order to identify where they are using RSA and ECC. Second, it will be important to understand what assets and communications are at risk in order to understand how to provide the right road map to migrate to postquantum cryptography.
2. Hybrid Encryption Systems: Another such step is to reach out for hybrid encryption systems so that organizations can move themselves out of the vulnerable spot created by quantum computers. These techniques embed both classical and post-quantum encryption algorithms. This will provide backward compatibility and continued security during the transition phase while the technology matures.
3. Engage in Standardization Processes: Since post-quantum cryptography is still in the development phase, it is an opportunity for organizations to participate in global standardization processes, for example, driven by NIST. In this context, businesses can be assured that they will know and be involved in these processes, adopting solutions that will be widely accepted and interoperable across industries.
4. Upgrade Key Management and Cryptographic Systems: With the dawn of quantum-safe encryption, organizations will be compelled to update key management in regard to newly presented quantum-resistant encryption algorithms, such as hardware security modules (HSMs) that support PQC and investments in quantum-safe hardware.
5. Intersectoral Collaboration Across Government and Industry: International cooperation will be a significant factor in this fight against the quantum menace. This would be so essential for creating global standards and solutions that can secure data in the quantum world, where governments, industries, and cybersecurity communities collaborate. This would ensure a uniform approach to the transition to quantum-safe encryption with the minimum chance of leaving behind security gaps.
Conclusion
Quantum computing is no more a dream but somewhat real, and the underlying potential to disrupt industries, especially in cybersecurity, is huge. While quantum computers hold immense promise, they also pose a great threat to the current methods of encryption that keep our digital world safe. It can break those algorithms at the heart of modern security, such as RSA and ECC, thus leaving sensitive data exposed.
Not all is lost, however, as the arrival of post-quantum cryptography and quantum-safe encryption, such as QKD, will help. The challenges, to say the least, are huge, notably scaling up these technologies and their introduction into existing systems. Work is ongoing but fundamental in preparing for the quantum computing world.
The message, therefore, is clear: cybersecurity professionals and organizations cannot afford to wait. Proactive planning, migration of systems to hybrid ones, and keeping themselves updated about post-quantum developments will be crucial in protecting data against the quantum threat. As we approach the quantum era, a certain balance between embracing its great potential and mitigating its risks will define the next phase of cybersecurity.
Subscribe to my newsletter
Read articles from Victor Uzoagba directly inside your inbox. Subscribe to the newsletter, and don't miss out.
Written by

Victor Uzoagba
Victor Uzoagba
I'm a seasoned technical writer specializing in Python programming. With a keen understanding of both the technical and creative aspects of technology, I write compelling and informative content that bridges the gap between complex programming concepts and readers of all levels. Passionate about coding and communication, I deliver insightful articles, tutorials, and documentation that empower developers to harness the full potential of technology.