Extraction and Purification of Membrane Proteins
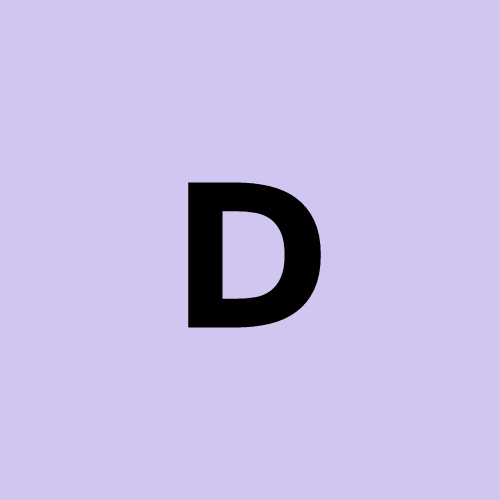
Membrane proteins play key roles in cellular functions, including regulation of ion transport across membranes, sensing and transmission of chemical and electronic signals, and regulation of cellular accessory functions. In addition, they are also important drug targets.
Membrane proteins can be classified into peripheral membrane proteins and integral membrane proteins based on how and to what extent they bind to the membrane. Peripheral membrane proteins are exposed on both sides of the lipid bilayer and are attached to the membrane surface by electrostatic interactions or hydrophobic interactions with weak binding. Integrated membrane proteins have at least one segment of protein sequence embedded in the biofilm and bind more strongly to the biofilm.
To understand the mechanism of biochemical reactions of membrane proteins and to design new drug targets, purification methods of membrane proteins are particularly important. Intact membrane proteins are more hydrophobic and tend to form precipitates during membrane lysis, complex purification processes lead to lower recovery of membrane proteins, and the membrane proteins themselves are lowly expressed in natural hosts. Therefore, it is important to select purification methods that not only extract membrane proteins efficiently, but also maintain the natural spatial structure and physicochemical properties of membrane protein molecules.
Extraction of Membrane Proteins
- Extraction of peripheral membrane proteins
The presence of hydrophobic and basic amino acids in the membrane binding sites of peripheral membrane proteins allows them to bind to biological membranes through the hydrophobic amino acids of peripheral membrane proteins with looser forces. Therefore, the isolation of peripheral membrane proteins requires only the disruption of the electrostatic force between them and the membrane, and not the complete disruption of the membrane. Peripheral membrane proteins can be dissociated from membranes using high salt, strong acid or strong base buffers. High salt buffers reduce electrostatic interactions between proteins and phospholipid head groups; strong acids or bases allow the separation of peripheral membrane proteins from intact membrane proteins by disrupting the closed membrane structure without disrupting the lipid bilayer. Dissociation using high ionic strength NaCl and KCl, alkaline or acidic buffers and metal chelators leads to significant separation between peripheral proteins and membranes, increasing the solubility of hydrophobic acidic residues in water and decreasing hydrophobic interactions. The dissociated peripheral membrane proteins are usually soluble in water and have similar properties to water-soluble proteins, and can be purified in the next step by methods similar to those used for water-soluble proteins.
- Extraction of integrated membrane proteins
Integrated membrane proteins consist of single or multiple transmembrane regions. Prior to purification, integral membrane proteins need to be dissociated from the lipid bilayer and become independent proteins. This process requires the use of a potent detergent to solubilize the integral membrane protein from the biofilm. The detergent solubilizes the protein by binding to the hydrophobic part of the integral membrane protein and interacting with the hydrophilic part of the protein. Commonly used detergents include Tween-80, sodium dodecyl sulfate, and cholate.
Purification of Membrane Proteins
After solubilization of membrane proteins using suitable detergents, the target proteins can be separated. Chromatographic techniques such as gel filtration, ion exchange, hydrophobic chromatography, affinity chromatography and sucrose density gradient centrifugation can be used for the purification of membrane proteins. Since certain detergents may affect the efficiency of downstream chromatographic techniques, the selection of a suitable purification method also needs to be considered depending on the type of detergent.
Gel Filtration
Gel filtration is the separation and purification of proteins using the difference in size of the protein molecules and the pore size of the preloaded column gel. A porous gel is used as the stationary phase. A gel column loaded with gel has two measurable liquid volumes.
Inner volume: the volume of liquid present in the voids inside the gel
External volume: the volume of liquid present between the gels
Progressive separation of molecules of different sizes by gel filtration (Prapulla et al., 2014)
Large molecules will flow out of the gel column first after passing through the outer water volume under the action of the mobile phase. Small molecules can enter the inner water volume of the gel and will be discharged from the gel column later, so the separation can be achieved. If a decontaminant is used for membrane proteins, the decontaminant must be present in both the sample buffer and the mobile phase.
Ion exchange
Biomolecules such as proteins usually have charge motifs on their surfaces. The charge on the surface of a protein depends on the isoelectric point of the protein itself and the pH of the environment in which it is placed. ion-exchange gels can be divided into cation-exchangers and anion-exchangers based on the difference in the ligands of the charges they carry. The ligands of cation exchange agents are negatively charged and can bind positively charged proteins. The ligand of the anion exchanger is positively charged and can bind negatively charged proteins. Depending on the strength of the protein charge binding ability, the target protein can be separated by elution using linear or specific pH or salt gradients.
Hydrophobic chromatography
Hydrophobic regions exist on the surface of macromolecules such as proteins that are able to couple with hydrophobic ligands in hydrophobic adsorbents. Using the hydrophobic microregions on the protein surface or the strength of the interaction between hydrophobic residues exposed to the molecular surface in a high salt environment and hydrophobic ligands in the stationary phase, components with weak to strong hydrophobic interactions can be separated in order from high to low ionic strength eluates. The high salt concentration interacts strongly with water molecules, leading to a reduction of water molecules forming cavities around hydrophobic molecules and promoting the binding between hydrophobic molecules and hydrophobic ligands of the medium.
Effect of salts on hydrophobic interactions (Kulkarni et al., 2022)
Affinity Chromatography
Affinity chromatography uses highly specific biological interactions between molecules as the basis for binding, specifically binding target proteins from complex mixtures such as antibodies and antigens, enzymes and substrates, and receptors and ligands. The stationary phases of affinity chromatography use general ligands (e.g. lectins), specific ligands (e.g. enzyme reactants) and antibodies, respectively. Currently, the following types of affinity chromatography are most commonly used.
- Lectin affinity chromatography, where lectins are sugar-binding proteins that can be used for the purification of membrane glycoproteins.
General workflow of immobilized-lectin affinity chromatography (Hashim et al., 2017)
Antibody affinity chromatography, based on the specific binding of antibodies and antigens.
Covalent affinity chromatography, which separates and purifies target proteins based on covalent interactions between the target protein and the ligand.
According to the properties of membrane proteins, the selection of suitable affinity chromatography ligands based on ligand-ligand binding is one of the most common and effective ways to purify membrane proteins today.
Sucrose density gradient centrifugation
After sucrose solution is centrifuged at high speed, a continuous or discontinuous density gradient is formed in the centrifuge tube, which can separate proteins with different relative molecular weights by gravity or centrifugal force. Because proteins have different molecular sizes and different densities, sucrose density gradient centrifugation enables fine purification of multi-component proteins. The process of detergent solubilization of membrane proteins is divided into several stages, and several different components such as detergent monomer, detergent micelles, lipid/protein/detergent, protein/detergent and lipid/detergent are present in the sample solution. All of them have different degrees of density magnitude, and the different component substances can be separated by sucrose density gradient centrifugation process.
Creative Proteomics provides membrane protein isolation and purification, identification and multi-omics analysis services to help obtain membrane protein products, as well as a wide range of protein quantification and other common downstream analyses.
References
S.G. Prapulla, N.G. Karanth, in Encyclopedia of Food Microbiology (Second Edition), 2014.
Kulkarni, R., & Bose, K. (2022). Protein Purification by Reversed Phase Chromatography and Hydrophobic Interaction Chromatography. In Textbook on Cloning, Expression and Purification of Recombinant Proteins (pp. 221-247). Springer, Singapore.
Hashim, O. H., Jayapalan, J. J., & Lee, C. S. (2017). Lectins: an effective tool for screening of potential cancer biomarkers. PeerJ, 5, e3784.
Subscribe to my newsletter
Read articles from dorawest1 directly inside your inbox. Subscribe to the newsletter, and don't miss out.
Written by
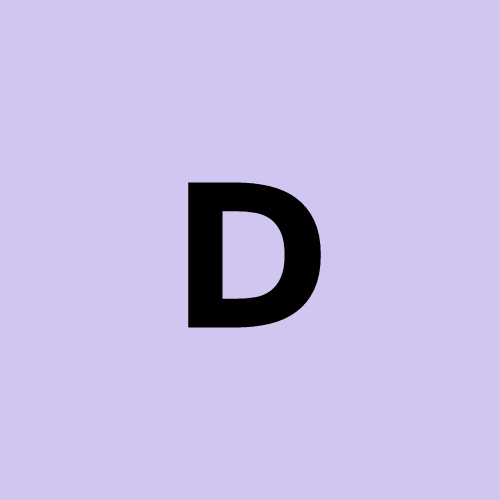