Laser Basics
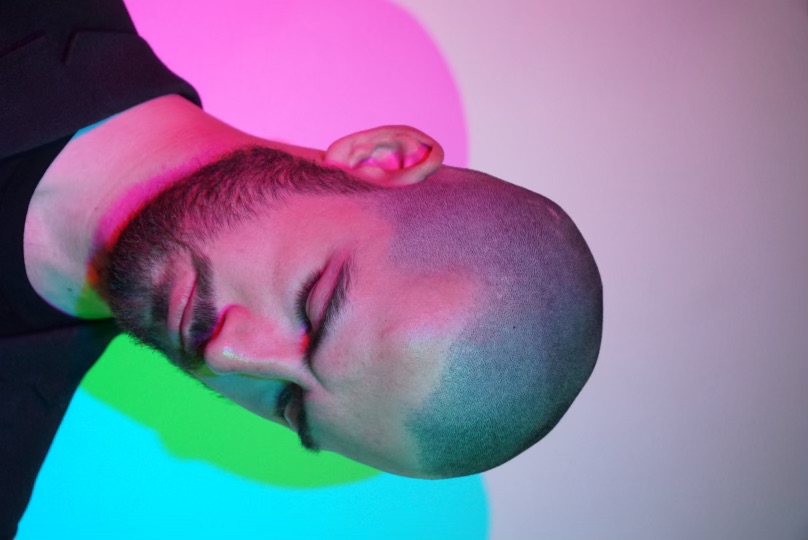
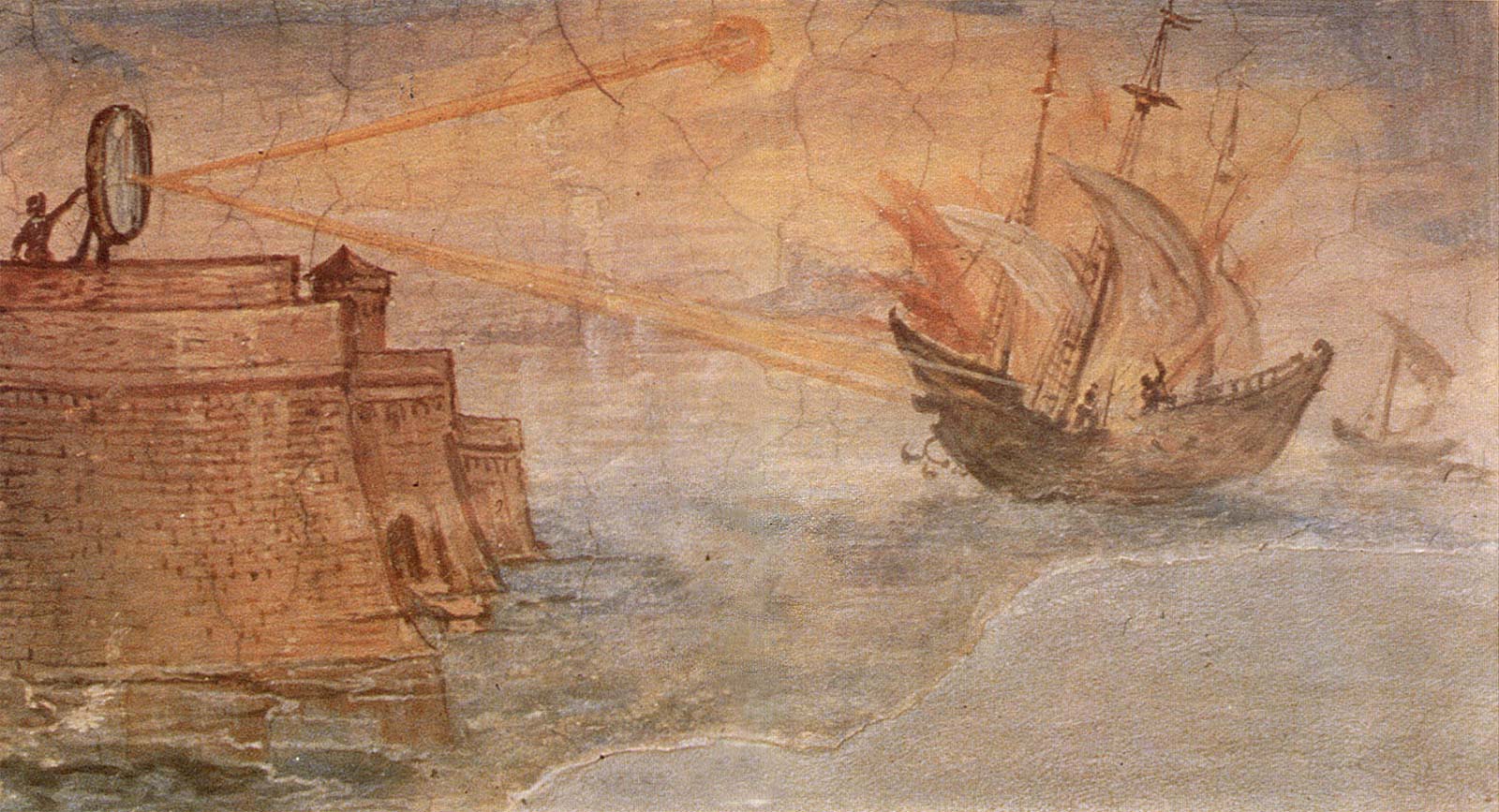
Although the first operational laser began in 1960, the very science of lasers began in 1917, where Albert Einstein reassessed Max Planck’s laws of radiation. Einstein’s Zur Quantentheorie der Strahlung (On the Quantum Theory of Radiation) lays the foundations of physics which we use to operate a laser. Laser (or Light Amplification by Emission of Stimulated Radiation) would be the pinnacle tool which would reshape every technological advancement of the 20th century.
By 1960, the first laser was operated by Theodore H. Maiman at Hughes Research Laboratories, Malibu. In 1960, however, the larger scientific community merely saw this phenomenon as a reflection of the children’s sci-fi comics which were popular at the time. Since then, however, the laser has been an immense game-changing in fields ranging from manufacturing, medicine, scientific, hobbyists and some of the most used technological inventions such as Blu-ray players and communication devices.
Basics
What does a LASER mean? L*ight **A*mplification means to make light brighter and more intense. One the mechanisms to do this is by *S*timulated *E**mission*, or triggered by another source.
Lasers which we are familiar with are typically radiation in the visible section of the electromagnetic spectrum. Lasers can also come in a variety of other colours, such as infrared, ultraviolet, X-Ray, and in theory you could build a gamma-ray laser –however this is extremely challenging due to the intense Doppler broadening of your spectral lines from thermal motion because of the intense load and heat generated onto the gain (we’ll discuss what gain means later).
All of these types of radiation are externally generated. Technically, the very first “laser” was a microwave amplified device, or “maser” which were stimulated by radio waves.
How does this amplification work? We are familiar with holding a microphone up to the connected speaker, whereby a feedback loop generates an amplified electrical signal which is emitted as sound, which is received and amplified as electrical signal, and so on. This amplification is nearly instantaneous.
Similar to a signal processing platform that amplifies your sound, a selected gain medium may also be used to amplify a light signal. The gain medium can be solid, a liquid, a gas or even a plasma. By selecting different gain media, we select different wavelengths of emitted light. The external stimulation is typically created by a basic light or electrical current. The energy moved into the gain media will change their populations at each energy level. Raising them to a higher energy level before relaxing to the ground level, emitting packets of energy, or photons of light. Every photon that is emitted from the gain medium will have the same polarisation, phase, energy and direction of travel: they are coherent.
What is stimulated emission? Within your gain medium, the physical atoms contain nuclei and electrons. Depending on the gain medium selected or designed, the stimulated emission (i.e. light or electricity) can change the energy state of the orbiting electrons. In the case of the gain media of the 785nm laser is GaAs crystal. When the GaAs crystal absorbs the energy, the atoms are elevated to an excited state of energy. While energy is being pumped into the crystal, more and more electrons are excited a higher energy level. Once more electrons are in the excited state than the ground state, creating a population inversion. Simply having a two-level excited-ground state system will not produce light. A four-energy level system is required, whereby the mediator state will produce light.
The electrons of the atoms will start to decay to a lower state, and loose energy in the form of an emitted photon of light. The energy of the photon decaying to the original state corresponds to the wavelength of light emitted in the laser.
Once a single excited atom of the crystal emits a photon, the photon passing its neighbouring excited atom will stimulate the emission of another photon. This photon is matched in phase, energy, polarisation and direction. These coherent photons will continue to stimulate other excited atoms to emit photons, leading to a cascade of emitted photons in the crystal and an increase in intensity.
Design
In order to generate even more amplification, two mirrors are placed at either end of the gain medium. Just like our microphone-speaker example, this system loops streams of coherent light, amplifying themselves and increasing the intensity. This set-up is called a laser resonator, whereby the two mirrors reflect laser light back and forth across the gain media, and generate more radiation.
To extract the trapped light out of the crystal, a small pinhole may be added to one of the mirrors—or one of the mirrors can be 1% transmissive. This light exits the device in a tightly focussed and reasonably collimated beam.
Selecting the gain medium will determine what colour or energy your laser light will be. The table below shows some popular gain media and their corresponding colours.
Laser Medium | Type | Wavelength (nm) | Colour/Region |
Ruby | Solid (Crystal) | 694.3 | Red |
Nd | Solid (Crystal) | 1064 | Infrared (IR) |
Argon-ion | Gas | 488, 514 | Blue-Green |
CO₂ | Gas | 10,600 | Far Infrared (FIR) |
Applications
There are several applications of lasers. Additive manufacturing also may be advanced by using a laser which hardens the melted resin when printing.
Some of the most useful laser-dependent technologies is not based on the laser’s ability to generate heat. Rather, it is based on the laser’s ability to transmit coherent and precisely directed signals. In territorial military operations, laser beams can be adjusted to emit invisible infrared light. This light is not visible to the target but is seen through the night-vision goggles, making it useful for military tactics.
Missile tracking technology may also make use of lasers, whereby a laser illuminates a target and the sensors of the missile make course corrections to direct the missile to the target.
When intersecting two or more coherent laser beams across a flowing region, an interference pattern is created. When a particle passes through this interference pattern, it passes and scatters regions of high and low intensities. This can be detected and analysed using a oscilloscope which shows a time-base of cyclical intensities, revealing the rate of flow across the pattern.
The Raman Effect
Raman spectroscopy could not be what it is today without the laser. The amplified directed light that composes the laser multiplied the intensity of the incident light which is necessary for the Raman effect. This has induced the Raman effect to higher orders of magnitude and reduces the exposure time necessary for a spectrum to be visible on a spectrograph. We owe it to the laser that we’re not waiting around to identify chemistry.
Subscribe to my newsletter
Read articles from Clark Gray directly inside your inbox. Subscribe to the newsletter, and don't miss out.
Written by
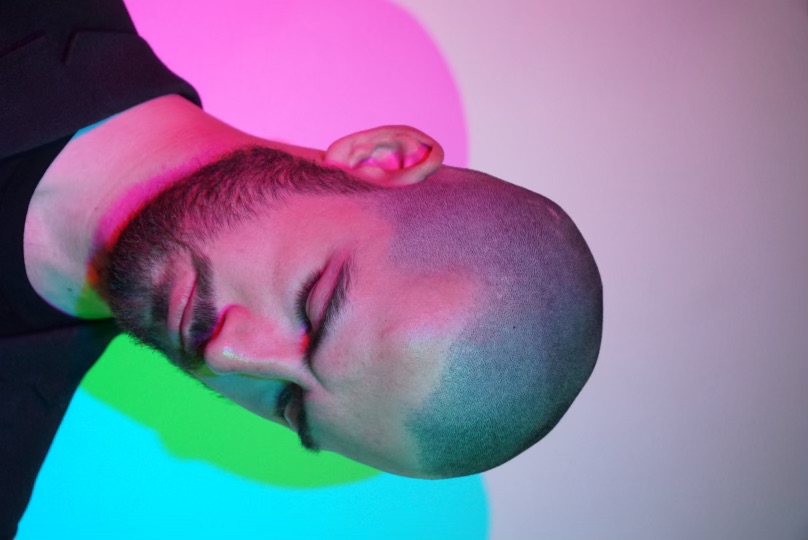